Proximal centriole loss and lateral head-neck attachment associatively evolved in the Muridae ancestor
The fan-shaped, atypical distal centriole was discovered in 2018 using advanced electron microscopy63; prior to this year, studies of spermatozoa mainly addressed the presence or lack of barrel-shaped centrioles. Two barrel-shaped, proximal and distal centrioles are found in the spermatozoa of most basal vertebrate external fertilizers37. In contrast, classic transmission electron microscopy (TEM) studies in mammalian spermatozoa found only one barrel-shaped centriole–the proximal centriole–with a few exceptions in marsupial and some rodent species that appear to lack it (Supplementary Fig. 1). Because rodent spermatozoan ultrastructure has undergone extensive phylogenetic study, and because the stereotypical proximal centriolar structure enables detection of evolutionary changes, we used rodent proximal centriolar ultrastructure to track the evolutionary changes leading to their disappearance. To this end, we systematically surveyed past TEM studies of rodent spermatozoan centrioles (Supplementary Fig. 1b, Supplementary Data 1).
The precise phylogeny of proximal centriole loss is unknown. In general, the literature on rodent sperm centrioles is confusing and at many times appears to claim that rodent spermatozoa lack a proximal centriole. For example, Manandhar and colleagues wrote that “rodent spermatozoa totally lack centrioles” p. 25666. Similarly, Xu and colleagues wrote that “spermatozoa of several species (e.g., rodents) lack centrioles due to complete centriolar degeneration” p. 20167. However, when we surveyed the original literature on rodent spermatozoan ultrastructure, we found that a proximal centriole was reported to be present in most rodents and in all three of the main rodent suborders (Supplementary Fig. 1b, Supplementary Data 1). A proximal centriole was reported in all studied rodent species of suborder Hystricomorpha, which includes the guinea pig (Cavia porcellus)68,69, chinchilla (Chinchilla lanigera)70, cane rat (Thryonomys swinderianus)71, agouti (Dasyprocta aguti)72, Spix’s yellow-toothed cavy (Galea spixii)73, and naked mole rat (Heterocephalus glaber)74. A proximal centriole was also reported in the rodent species of suborder Sciuromorpha, which includes the Korean squirrel (Tamias sibiricus)75 and the flying squirrel (Glaucomys volans)76. Finally, a proximal centriole was reported in three studied species of family Cricetidae of suborder Myomorpha: golden hamster (Mesocricetus auratus)77, Chinese hamster (Cricetulus griseus)78, and Winkelmann’s mouse (Peromyscus winkelmamzi)79. The presence of a proximal centriole in the three main rodent suborders suggests that a canonical proximal centriole was present in the last common rodent ancestor and is present in many existing rodent species.
In contrast, a proximal centriole is reported to be absent in the five studied members of family Muridae of suborder Myomorpha, which includes the house mouse (Mus musculus)24,56, rat (Rattus norvegicus)43, Mongolian gerbil (Meriones unguiculatus), and two Apodemus species (Apodemus agrarius coreae and Apodemus speciosus peninsulae)80,81. The location of the missing proximal centriole in the spermatozoan neck of these species is marked by an empty, vault-like space, suggesting that a proximal centriolar remnant may be present. Indeed, a recent study using state-of-the-art cryo-electron microscopy in house mouse spermatozoa confirmed that there were some microtubule remnants where the proximal centriole should be located56. The absence of a proximal centriole in multiple genera of family Muridae contrasted with its presence in multiple genera of family Cricetidae suggests that proximal centriole degeneration occurred after the divergence of Muridae from Cricetidae, which happened about 20 million years ago82.
Proximal centriole loss correlates with lateral tail attachment to the head in the Muridae ancestor
The observation that a barrel-shaped proximal centriole is present in Cricetidae despite its dispensability post-fertilization suggests that the proximal centriole has a function in the cricetid spermatozoon. Similarly, the observed absence of a barrel-shaped proximal centriole in Muridae, despite its presence in Cricetidae, suggests that the proximal centriole became dispensable in the murid spermatozoon. Since the sperm centriole connects the tail to the head as part of the head-tail connecting apparatus (HTCA)83 and can coordinate sperm tail and head movement13, we considered the structure of the proximal centriole in the context of its function in sperm neck and head morphology. Mammalian spermatozoa possess the same basic structural components (head, neck, and tail) but can vary in their morphology, size, and, particularly, in their head shape and the location of the head-neck junction.
Most Eutherian mammals and, specifically, rodents of suborders Hystricomorpha and Sciuromora have a short, oval- or paddle-shaped sperm head with a sperm neck that is attached at or near the center of the head (referred to as a centrally inserted neck)84 (Fig. 1). As a result, it is thought that the last common rodent ancestor had a paddle-shaped sperm head with a centrally inserted neck85,86. Rodent species of suborders Hystricomorpha and Sciuromora also have a proximal centriole, suggesting that these three characteristics (i.e., paddle-shaped head, centrally inserted neck, and the proximal centriole) are associated with each other (Fig. 1c).
While several groups of Myomorpha have the ancestral, paddle-shaped head and centrally inserted neck (i.e., tail attached below the head base center), some have evolved a unique sperm head shape and head-neck attachment point86,87. For example, the last ancestor of Cricetidae and Muridae is thought to have evolved a novel, elongated, sickle-shaped head86 (Fig. 1c). Many species of family Cricetidae have a centrally inserted or off-center head-neck attachment (i.e., tail attached asymmetrically, below the head base)85,86 and a proximal centriole, suggesting that their proximal centriole was maintained after the appearance of a sickle-shaped head77,78,79. Therefore, in these cricetids, the presence of a proximal centriole and a centrally inserted neck correlate with each other.
As discussed above, the proximal centriole is absent in family Muridae, and many species in this family exhibit, in addition to a sickle-shaped head, a unique, lateral, head-neck attachment (i.e., tail attached to the side of the head, parallel to the base) (Fig. 1d–e). The difference between the head-neck attachment in Cricetidae and Muridae species suggests that, in rodents, a proximal centriole is present when the neck is centrally inserted (the ancestral form) or off-center and that a proximal centriole is absent when the neck is laterally attached to the head (the derived form), as in Muridae. Therefore, we hypothesize that centrioles are structurally degenerated as part of the evolution of the lateral head-neck attachment in Muridae.
The primary structure of FAM161A evolved in Muridae and Cricetidae
The spermatozoa of some rodent clades have evolved to successfully fertilize without centrioles. The molecular basis underlying this evolutionary change is unknown, but we hypothesized that it is associated with a change in centrosomal protein structure and function. To identify candidate proteins, we searched for sperm centrosomal proteins that diverge specifically in house mice in contrast to other mammals. We identified sperm centrosomal proteins by comparing the spermatozoan proteome88,89,90,91 to the centrosomal protein database92. We performed a rapid and simple protein sequence (i.e., primary structure) comparison between humans and bovine, both of which have two spermatozoan centrioles, and house mice, which lack spermatozoan centrioles; we refer to this calculation as the Identity Ratio (Fig. 2a). A ratio of 1.00 indicates that the human and house mouse orthologs have a percent amino acid identity equal to the ratio between human and bovine; a ratio of more than 1.00 indicates that the human and house mouse orthologs have a greater percent primary structure identity than the ratio between human and bovine; and a ratio of less than 1.00 indicates that the human and house mouse orthologs have a lower percent primary structure identity than the ratio between human and bovine. According to simple phylogenetic considerations (Fig. 2b) and assuming a constant evolutionary rate, we would expect humans to be more identical to house mice than to bovine and, consequently, that their proteins should have an Identity Ratio greater than 1.00.
a Calculated extended identity ratios. Hs Homo sapiens, Mm Mus musculus, Bt Bos taurus, Oc Oryctolagus cuniculus. b Phylogenetic tree showing the evolutionary position of the four mammals used in the extended identity ratio calculations and their proximal (PC) and distal (DC) centriolar structures82. c The top 10 identity ratio (IR) hits and their extended identity ratios (EIR). Extended identity ratios were calculated for proteins near the FAM161A genomic location (d), proteins influenced by sperm competition (e), and sperm distal centriolar proteins (f). Rodent phylogenetic tree (g) with FAM161A sequence identity relative to human FAM161A (h). Percent identity is also shown as average ± SD for individual clades. *P < 0.05, **P < 0.01 (unpaired, two-tailed t test; exact p-values are provided in the figure and Source Data File); ns not significant. (i) Bayesian phylogeny of FAM161A inferred using nucleotide sequences of mammalian species. (j) Bayesian phylogeny of FAM161A inferred using nucleotide sequences of Myomorpha species. The scale bars in i and j represent the number of nucleotide substitutions per site.
We found that 450 putative sperm centrosomal proteins had an Identity Ratio range between 0.63 and 1.90, with most centrosomal proteins having an Identity Ratio below 1.00 (a median of 0.99 with a lower 1.5 interquartile of 0.91) (Supplementary Data 2). This low Identity Ratio suggests that mouse centrosomal protein sequences change slightly more than those of bovines and is consistent with previous observations of mutational rate acceleration in the Muridae lineage93. Most significantly, FAM161A, a known component of the sperm atypical centriole that exhibits species-specific labeling differences13, had the third lowest Identity Ratio (0.74) in this list and was far below the lower 1.5 interquartile, suggesting that FAM161A has extensively evolved in house mice (Fig. 2c). To be more stringent in quantifying the level of specific change in house mouse protein sequences, we calculated an Extended Identity Ratio (EIR), which compares the three sequence identity values between house mice and either of three species (humans, bovines, or rabbits) possessing two spermatozoan centrioles to the three sequence identity values between the same species (humans, bovines, and rabbits) (Fig. 2a). Using this quantification, we found an EIR of 0.77 for FAM161A, further supporting its potential role in sperm centriole evolution.
Reproductive genes evolve faster than other genes due to adaptive diversification associated with sperm competition, cryptic female choice, and sexual conflict94,95. In contrast, genes integral to sperm function evolve faster in monogamous species than in promiscuous species, possibly because of a reduced selective constraint96. Because house mice exhibit a high level of gene sequence diversification93, the above evolutionary factors may result in a shift in the EIR of FAM161A to below 1.00. Therefore, we tested whether other contributory evolutionary trends drive the low EIR of FAM161A. In mammals, FAM161A has a paralog, FAM161B, with a higher EIR (EIR = 1.04). Proteins whose genes flank the Fam161a gene in house mouse chromosome 11 also had higher EIR (EIR = 0.84–1.02) (Fig. 2d). These values suggest that the Fam161a gene evolved without haplotype selection and is an evolutionary hotspot. Finally, sperm proteins known to have divergent sequences also had higher EIR (EIR = 0.88–1.00) (Fig. 2e)97. Altogether, these comparatively higher EIR values suggest that the FAM161A sequence selectively evolved in house mice.
FAM161A belongs to a small set of centriolar inner scaffold proteins that form a unique rod structure in the atypical distal centriole. Yet, the set’s other proteins, such as POC1B and POC5, had higher EIR (EIR = 0.91–1.01) than FAM161A (Fig. 2f). Similar results were observed by calculating dN/dS ratio (ω). In this analysis, ω > 1 indicates positive (adaptive, diversifying, increasing amino-acid diversity) selection, ω = 1 indicates neutral evolution, and ω < 1 indicates negative (purifying, the selective removal of deleterious mutations) selection. The median of mammalian protein ω values falls between 0.08 and 0.10, depending on the species being compared98. We expected that most centriolar rod proteins would be under purifying selection because centrioles are essential in animal development and physiology23,99. Indeed, we found that ω of rodent POC1B and POC5 were close to the mammalian median (ω = 0.19–0.27) (Supplementary Table 1). In contrast, FAM161A had a much higher ω of 0.58, suggesting that the FAM161A sequence is evolving more quickly than other distal centriolar proteins. Consistent with our previous EIR analysis, the ω value of FAM161A was higher in Muridae than in Cricetidae and other Myomorpha species (0.63 > 0.57 > 0.49, respectively), supporting our conclusion that it is rapidly evolving.
To understand the evolution of FAM161A in mammals, we analyzed the FAM161A primary structure identity of humans to other species with a predicted proteome in the National Center for Biotechnology Information (NCBI) protein database (Fig. 2g–h). Human FAM161A has a 70–77% primary structure identity to bovines and rabbits. A similar primary structure identity to human FAM161A was found in species from suborder Sciuromorpha (76 ± 0.6), suborder Hystricomorpha (71 ± 3), and the non-Muridae Cricetidae clades of suborder Myomorpha (69 ± 4). This primary structure identity suggests that basal rodents with centriole-dependent embryonic development, a proximal centriole, and centrally inserted neck have similar FAM161A rates of evolution. In contrast, in the Muridae-Cricetidae clades, FAM161A showed a lower primary structure identity to human FAM161A, at 60 ± 0.2 in Cricetidae species and 57 ± 2 in Muridae species. This statistically significant difference suggests that the FAM161A primary structure started evolving in the Muridae and Cricetidae ancestor (which had off-center and lateral head-neck attachment) and accelerated in the Muridae lineage (species that have centriole-independent embryonic development in common). Considering the other sperm changes (Fig. 1), these findings are consistent with the hypothesis that house mouse FAM161A underwent a distinctive primary structural change that correlates with the emergence of centriole-independent embryo development, lateral head-neck attachment, and proximal centriole loss.
Next, we generated a FAM161A phylogenetic tree of 115 mammals using MrBayes and IQtree (maximum-likelihood and Bayesian phylogenetic trees) (Fig. 2i) and found that the tree had eight main branches. Three large, related branches of non-rodent mammals included primates, carnivora, and ungulates, and exhibited relatively high FAM161A primary structural similarity (branches 1–3 in Fig. 2i). The remaining five branches comprised rodent species (branches 4–8 in Fig. 2i). One basal rodent branch included Muridae and Cricetidae species and was the most diverse group, as expected from accelerated adaptive evolution (branch 8 in Fig. 2i). The remaining four rodent branches were intermediate between non-rodent mammals and Muridae and Cricetidae species (branches 4–7 in Fig. 2i). Four different methods (Mixed Effects Model of Evolution, MEME; Fixed Effects Likelihood, FEL; Phylogenetic Analysis by Maximum Likelihood, PMAL; and Fast Unconstrained Bayesian AppRoximation, FUBAR) identified many more sites that have undergone adaptive evolution in rodents than in primates, carnivora, and ungulates (Supplementary Table 2). For example, PMAL and FUBAR identified greater than three-fold more positively and negatively selected sites in rodents than in primates, carnivora, and ungulates.
Furthermore, we searched for the rodent subgroup that drives accelerated adaptive evolution in rodents. We generated a FAM161A molecular tree in rodents using FEL (Fig. 2j) and found that the tree has three main branches, which corresponded to Muridae, Cricetidae, and other Myomorpha species. FEL found a similar number of positively selected sites in the three groups (14, 14, and 15 sites, respectively, P < 0.05) but a much smaller number of negatively selected sites in Muridae than in Cricetidae and other Myomorpha species (4, 87, and 48, respectively) (Supplementary Table 3).
Muridae testes express an evolutionarily novel FAM161A protein and mRNA isoform
Mammalian FAM161A has multiple predicted isoforms in the NCBI protein database. Isoforms of two different lengths were studied in humans and house mice100,101,102 (Fig. 3a, type 1 and type 2). Here, we refer to the long isoform, comprised of exons 1–7 and expressed in photoreceptors, as type 1 (house mouse 80 KDa, 700 aa, XP_006514891; human 83 KDa, 716 aa, NP_001188472). We refer to the shorter isoform, comprised of exons 1–3 and 5–7 and expressed ubiquitously, as type 2 (house mouse 74 KDa, 644 aa, XP_006514893; human 76 KDa, 660 aa, NP_001188472).
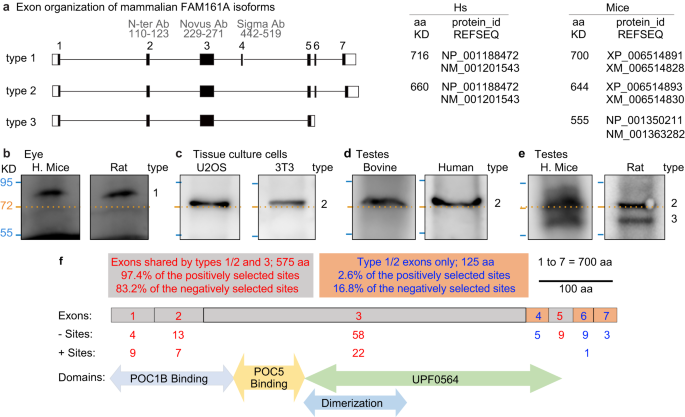
a Exon organization of FAM161A isoform types 1, 2, and 3. ID of predicted FAM161A isoforms and corresponding numbers of amino acids in humans and house mice. b–f Western blot analysis of FAM161A in house mouse and rat eyes (b), U2OS and 3T3 cells (c), bovine and human testes (d), and house mouse and rat testes (e). Numbers to the right of each blot pair indicate FAM161A isoform types. f Diagrammatic representation of Mus musculus FAM161A with protein domains, positively (+) selected sites identified by CodeML M8 modeling, and negatively (−) selected sites identified by FEL. All western blot images shown are representative of at least three independent experiments.
We studied FAM161A in tissue samples by western blot analysis. As expected, house mouse and rat eyes expressed an ~80 KDa protein, corresponding to the FAM161A type 1 isoform (Fig. 3b). Also, expectedly, we observed a single FAM161A isoform of molecular size just above ~72 KDa, probably corresponding to FAM161A type 2, in human and house mouse cells (i.e., U2OS and 3T3) (Fig. 3c). Similarly, in bovine and human testes, we found a single isoform just above ~72 KDa, expected to be FAM161A type 2 (Fig. 3d). These observations confirmed expectations based on published literature103.
Interestingly, in murids (e.g., house mice and rats), two FAM161A isoforms were expressed in the testes: one at ~72 KDa, which may correspond to type 2, and one shorter isoform below the 72 KDa marker, at ~60 KDa, which we refer to as the type 3 isoform (Fig. 3e). Analysis of house mouse spermatid mRNA shotgun sequencing data predicted that house mouse testes express four transcripts: a most common transcript, NP_001350211, coding for a 555-aa isoform, and an additional three minor transcripts, including XP_006514893, the type 2, 644-aa isoform (Supplementary Fig. 2)104. The new, 555-aa isoform has a predicted molecular weight of 64 KDa and is expected to be the type 3 isoform. It contains exons 1, 2, 3, 5, and the beginning of intron 5, which codes for five new amino acids, VVFIGX, followed by a unique, 3′ untranslated sequence (Fig. 3a). We refer to this unique exon 5 as exon 5′. Using 5′ RACE, PCR amplification, and sequencing, we confirmed that the type 3 and type 2 isoforms are expressed in house mouse testes (Supplementary Fig. 2b, c). The type 3 isoform was further confirmed by a search of the NCBI house mouse EST database, which found seven additional FAM161A transcripts with the unique type 3 C-terminus sequence in house mouse testes (Supplementary Fig. 2d).
The FAM161A type 3 isoform exhibits a relatively high level of positively selected sites
The differential expression of FAM161A isoforms may have resulted in a distinctive rate of evolutionary change. The longest isoform of FAM161A (type 1) has seven exons (700 aa in house mice): exons 1, 2, 3, and 5 (575 aa, or 82.1% of the protein) are found in all isoforms, whereas exons 4, 6, and 7 (125 aa, or 17.9% of the protein) are found only in non-type 3 isoforms. To identify which of these exons are under selection, we employed MEME to identify the sites that are under episodic diversifying selection across the four major mammalian groups (Fig. 3f).
We found that exons 4, 6, and 7 had 17 negatively selected sites out of 125 aa, a rate of 13.6% (Fig. 3f). Similarly, exons 1, 2, 3, and 5 had 84 negative sites out of 575 aa, a rate of 14.6 %. These similar rates suggest a similar level of purifying selection in type 1, 2, and 3 isoforms. In contrast, exons 4, 6, and 7 had only 1 positively selected site out of 125, a rate of 0.8%, whereas exons 1, 2, 3, and 5 had 38 positively selected sites out of 575 aa, a rate of 6.6%. This difference in rates is statistically significant, based on the Z Score Calculator for 2 Population Proportions (P = 0.01). The observation that relatively more positive selection occurs in the exons shared between types 1, 2, and 3 than in the exons not expressed in type 3 suggests that the type 3 isoform evolved faster than the other isoforms. Finally, Mus and Rattus species had few positively (17 and 18, respectively) and negatively (1 and 0, respectively) selected sites as identified by FEL, suggesting that FAM161A changes are fixed and stable in these genera.
House mouse and human FAM161A isoforms can localize to canonical centrioles
FAM161A is a centriolar protein, and its overexpressed human isoform localizes to the centriole in cultured cells105. Therefore, we investigated the localization of overexpressed human type 2, mouse type 2, and mouse type 3 to the centriole in human U2OS and mouse 3T3 cells by labeling the cells with antibodies that recognize centrosomal proteins106. Untransfected UTOS cells had one or a pair of pericentrin-labeled foci per cell (21% and 79% of the cells, respectively; N = 39). Cells expressing human type 2, mouse type 2, and mouse type 3 FAM161A had FAM161A in most pericentrin-labeled centrioles (Supplementary Fig. 3a, b). Similar results were observed when γ-tubulin marked the centrosome in U2OS cells (Supplementary Fig. 3c, d) or in 3T3 cells labeled against pericentrin (Supplementary Fig. 4a, b) or γ-tubulin (Supplementary Fig. 4c, d). However, in some cases, mouse type 3 had a lower rate of localization to the centrosome (Supplementary Fig. 4d). This finding suggests that, despite the differences in the primary structures of the three isoforms, they are all able to localize to canonical centrioles, although with some differences.
Because FAM161A has different localization patterns in the sperm atypical centriole and canonical centrioles, we also investigated protein interaction outside the canonical centriole by overexpression: see below.
Human and house mouse FAM161A isoforms have different microtubule and POC5 interactions
Human FAM161A type 1 interacts with human POC5 and POC1B64, which also interact with each other64. Human POC5 also interacts with human CETN protein family members107. Of these four proteins, only FAM161A interacted directly with microtubules (via amino acids 230–543 of type 2, domain UPF0564) (Supplementary Fig. 5a)105. Using the FAM161A-microtubule interaction in U2OS cells, we mapped binding between human FAM161A type 2 and POC5 or POC1B through their recruitment to the cellular microtubule network when co-expressed with FAM161A (Supplementary Fig. 5b–f). We complemented our protein interaction mapping with yeast two-hybrid assays and found that amino acids 1–141 of human FAM161A type 2 interacted with POC1B (Supplementary Fig. 5d), amino acids 141–230 of human FAM161A type 2 interacted with human POC5 (Supplementary Fig. 5c–d), and amino acids 1–222 of the human POC5 N-terminus interacted with amino acids 365–478 of the POC1B C-terminus (Supplementary Fig. 5e). These findings suggest a web of interactions between centriolar inner scaffold/rod proteins (Supplementary Fig. 5f).
House mouse and human FAM161A have distinct subcellular localization patterns to the microtubule cytoskeleton
The interacting domains of FAM161A include most of the positively selected sites in rodents (Fig. 2j). To test for potential functional differences due to divergent primary structure, we expressed house mouse and human FAM161A type 2 in human U2OS cells (Fig. 4). As expected, we found that both proteins localized to the microtubules (Fig. 4a), though they exhibited differences in their subcellular localization patterns and the extent of microtubule co-localization. We have distinguished four types of subcellular localization pattern: “cytoplasmic,” with linear FAM161A throughout the cytoplasm; “intranuclear,” with FAM161A foci in the nucleus; “perinuclear” (circular), with FAM161A around the nucleus; and “mix intranuclear-perinuclear”, characterized by both intranuclear and perinuclear patterns (Fig. 4a). Human FAM161A type 2 mostly localized to the cytoplasmic microtubules. In contrast, house mouse FAM161A type 2 showed a mostly intranuclear or mix intranuclear-perinuclear pattern (Fig. 4a, b). A similar difference in subcellular localization pattern was observed when the two FAM161A type 2 orthologs were expressed in mouse 3T3 cells (Supplementary Fig. 6). These distinct localization patterns suggest that the amino acid differences between human and house mouse FAM161A type 2 produce functional differences.
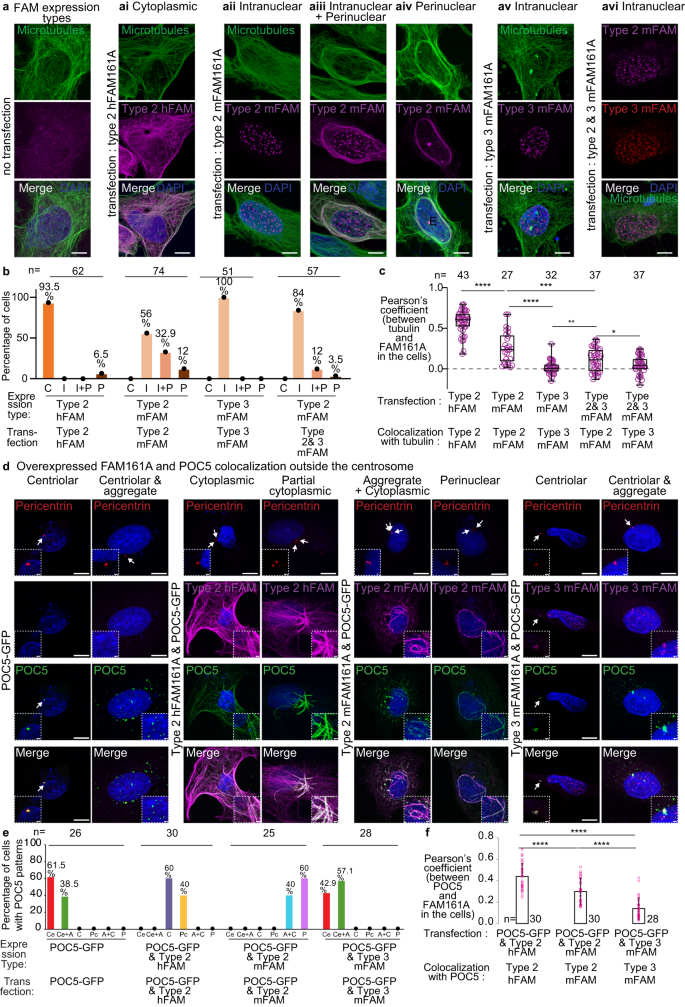
a Expression analysis of human FAM161A (hFAM161A) type 2 (second panel from left), house mouse FAM161A (mFAM161A) type 2 (middle three panels), mFAM161A type 3 (second panel from right), and the combination of mFAM161A types 2 and 3 (right panel) in U2OS cells. b Quantification showing the percentage of cells exhibiting each of the various expression patterns observed during expression analysis of hFAM161A type 2, mFAM161A type 2, mFAM161A type 3, and the combination of mFAM161A types 2 and 3. C, “Cytoplasmic”; I, “Intranuclear”; I + P, “Mix intranuclear-perinuclear”; P, “Perinuclear”. c Quantification of FAM161A colocalization with tubulin. ****P < 0.0001, ***P < 0.001, **P < 0.01, *P < 0.05 (unpaired, two-tailed t test; exact p-values are provided in the Source Data File); ns not significant, n number of cells, scale bars are 8 µm. The data shown are the representative images and compiled quantification from three independent experiments. Data are presented as box and whisker plots, where upper and lower bounds show interquartile range, the line within the box shows the median, and whiskers show minimum and maximum data points. d Co-overexpression of FAM161A isoforms and POC5 in U2OS cells. The inset in the bottom left corner shows a zoomed view of the site of the centriole, and the inset in the bottom right corner shows a zoomed view of non-centriolar POC5 locations in the cell. Scale bars are 8 µm, inset scale bar is 1 µm. e Quantification showing the percentage of cells exhibiting the various expression patterns observed during expression analysis of hFAM161A type 2, mFAM161A type 2, and mFAM161A type 3. Ce, “Centriolar”; Ce+A, “Centriolar + Aggregate”; C, “Cytoplasmic”; Pc, “Partial Cytoplasmic”; A + C, “Aggregate + Cytoplasmic”; P, “Perinuclear”. f Quantification of FAM161A colocalization with POC5. Statistical analysis used was unpaired, two-tailed t test. Source data are provided in the Source Data File.
FAM161A type 3 is 555 amino acids in length and composed of the homologous sequences that mediate binding to microtubules (type 3 amino acids 226–532, domain UPF0564), POC1B (type 3 amino acids 1–139), and POC5 (type 3 amino acids 140–226); however, it is missing the 95 amino acids encoded by type 2 exons 5 and 6, which have unknown function (Supplementary Fig. 7). In contrast to type 2, type 3 mostly formed foci in the nucleus of human U2OS cells (Fig. 4a, b) and mouse 3T3 cells (Supplementary Fig. 6). This localization pattern prompts the hypothesis that the protein domain differences between house mouse FAM161A types 2 and 3 produce functional differences.
Overexpressed mouse FAM161A type 3 has lower colocalization with overexpressed POC5 outside the centrosomes
RNAi-mediated FAM161A knockdown in U2OS cells had a small effect on centrosomal POC5 localization, suggesting that FAM161A has limited control on POC5 recruitment to the canonical centrioles108. Consistent with that, we found that POC5 localized to canonical centrioles when each of the three FAM161A isoforms were overexpressed (human type 2: 100%, n = 30; mouse type 2: 100%, n = 30; mouse type 3: 100%, n = 25) (Supplementary Fig. 8).
Overexpressed POC5 created aggregates in ~39% of transfected cells (Fig. 4d, e). As expected, when human FAM161A type 2 was co-overexpressed with POC5, the POC5 aggregates disappeared in all cells (N = 30). Instead, POC5 appeared along the microtubules with FAM161A (“Cytoplasmic,” in 60% of transfected cells) and, sometimes, along only some of the microtubules (“Partial cytoplasmic,” in 40% of transfected cells) (Fig. 4d, e). However, when mouse FAM161A type 2 was co-overexpressed with POC5, the POC5 colocalized with FAM161A in the aggregates and cytoplasm (in 40% of transfected cells) as well as around the nucleus (in 60% of transfected cells). Interestingly, when mouse type 3 was co-overexpressed with POC5, the POC5 colocalized with FAM161A in the centrosomes, but only 50% of POC5 aggregates contained FAM161A (N aggregates = 497, N cells = 12). We quantified overexpressed POC5 colocalization with various FAM161A isoforms and found that POC5 colocalized significantly less with mouse type 3 FAM161A than with overexpressed human and mouse type 2 (Fig. 4f). This reduced colocalization suggests that mouse type 3 has a weaker interaction with POC5 than mouse type 2.
Overexpressed mouse FAM161A type 3 can prevent overexpressed FAM161A type 2 and POC1B from localizing to the cytoplasmic microtubules
Human FAM161A type 2 forms homodimers via amino acids 230–386105. This amino acid sequence corresponds to amino acids 226–380 of house mouse types 2 and 3, suggesting that they can also dimerize. To gain insight into the effects of house mouse types 2 and 3 on each other, we co-expressed them in U2OS cells. We found that type 3 prevented type 2 from colocalizing with the circular microtubules (perinuclear pattern) and, instead, targeted type 2 to foci in the nucleus (intranuclear pattern) (Fig. 4a–c). This observation suggests that mouse FAM161A type 3 can act as a dominant negative isoform that prevents FAM161A type 2 from localizing to the cytoplasmic microtubules.
Human FAM161A type 2 interacts with POC1B and recruits it to the cytoplasmic microtubules109. Therefore, we next examined the interaction of house mouse FAM161A with mCherry-POC1B by coexpressing them in U2OS cells. Usually, rod proteins (i.e., POC1B, POC5) form aggregates when expressed in U2OS cells (without expressing FAM161A), because these proteins interact with each other (Supplementary Fig. 5a). As expected, we found that house mouse FAM161A type 2 recruited POC1B to the microtubules (Supplementary Fig. 9a). However, FAM161A type 3 did not recruit POC1B to the microtubules but instead colocalized with POC1B aggregates (Supplementary Fig. 9b–e). This suggests that both isoforms maintained the ability to interact with POC1B, which is expected, since the FAM161A N-terminal domain that mediates the interaction with POC1B is found in both isoforms (Supplementary Fig. 5f). Finally, co-expressing type 2 with type 3 inhibited type 2 from recruiting POC1B to the microtubules (Supplementary Fig. 9c). These observations suggest that FAM161A type 3 can interact with both FAM161A type 2 and POC1B but specifically lost the ability to interact with microtubules and may act as a dominant negative in vivo. Altogether, the above in vitro observations suggest that house mouse testes express FAM161A proteins that localize to canonical centrioles but are functionally distinct from human FAM161A.
Cricetidae and Muridae spermatozoan FAM161A is present only in the distal centriole
FAM161A is a component of the distal and proximal centrioles in basal mammals, such as humans, bovines, rabbits, and dogs13 (Supplementary Fig. 10). To gain insight into the localization of FAM161A in rodents, we stained mature spermatozoa isolated from the caudal epididymis of house mice, Peromyscus maniculatus (deer mouse; family Cricetidae, subgroup Neotominae), and Microtus ochrogaster (prairie vole; family Cricetidae, subgroup Arvicolinae) using FAM161A Ab1, POC1B Ab2, and tubulin antibodies (Fig. 5a–c).
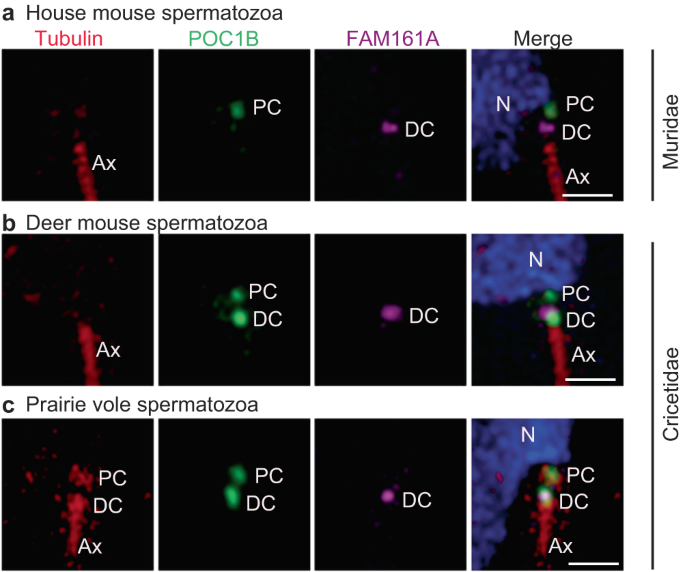
Rod protein localization in mature spermatozoan necks of house mice (a), deer mice (b), and prairie voles (c). Tubulin is used as a marker for the centriole and axoneme. PC proximal centriole, DC distal centriole, Ax axoneme. Scale bars are 1 µm. The images shown are representative of three independent experiments.
In the house mouse spermatozoan neck, FAM161A labeled a single focus near the axoneme tip, which most likely represents a distal centriolar remnant (Fig. 5a). The FAM161A focus was uniquely short and wide, unlike in humans, bovines, and rabbits, where it is longer, indicating a distinct localized distribution. FAM161A and tubulin labeling were undetected in the proximal centriole (Fig. 5a). POC1B labeled a single focus proximal to the nucleus that most likely corresponds to the proximal centriolar remnant. This data suggests that despite centriolar ultrastructural loss in house mice, some centriolar inner scaffold proteins are still present, though in a unique pattern.
Like in house mice, in deer mice, FAM161A labeled a single, short, and wide focus on the distal centriole, indicating that house mice and deer mice have similar FAM161A localization patterns (Fig. 5b). Also, like in house mice, tubulin was not detected in the neck of deer mouse sperm. However, in contrast to house mice, POC1B in deer mice distinctly labeled two foci, as observed in most mammals, which we believe represent the proximal and distal centrioles. Overall, FAM161A and POC1B localization in deer mice show characteristics that are intermediate between most mammals and house mice.
Finally, in prairie voles, which belong to a different Cricetidae subgroup, tubulin was detected in the two spermatozoan centrioles, and FAM161A labeling was similar to that of deer mice and house mice (i.e., showing a wide focus on the distal centriole) (Fig. 5c). Overall, FAM161A shows an evolutionarily novel, single-focus labeling pattern in Muridae and Cricetidae, suggesting that a change in FAM161A localization took place in the common ancestor of these families. Also, these findings support the hypothesis that sperm centrioles gradually evolved in the superfamily Muroidea and that proximal centriole degeneration is most extreme in the family Muridae.
Humans, rabbits, and bovines share a conserved centriole remodeling program
Mammalian spermatozoan centrioles are remodeled from canonical proximal and distal centrioles during spermiogenesis in the seminiferous tubule of the testes24,63. To study FAM161A changes during mammalian evolution, we first studied its localization relative to three other typical centriolar inner scaffold and atypical distal centriolar rod proteins (CETN1, POC5, and POC1B) in basal mammals (with two spermatozoan centrioles), namely humans, rabbits, and bovines. To do this, we performed immunofluorescence (Fig. 6a–c) and quantified the total centriolar relative labeling intensities (Fig. 6d–f). We looked at three sperm cell stages: pre-haploid sperm (spermatogonia and spermatocytes), round spermatids, and elongated spermatids. These stages were identified based on the location of cells relative to basal lamina in the seminiferous tubules, staining with lectin PNA, and the shape of the nucleus (Fig. 6ai, bi, ci).
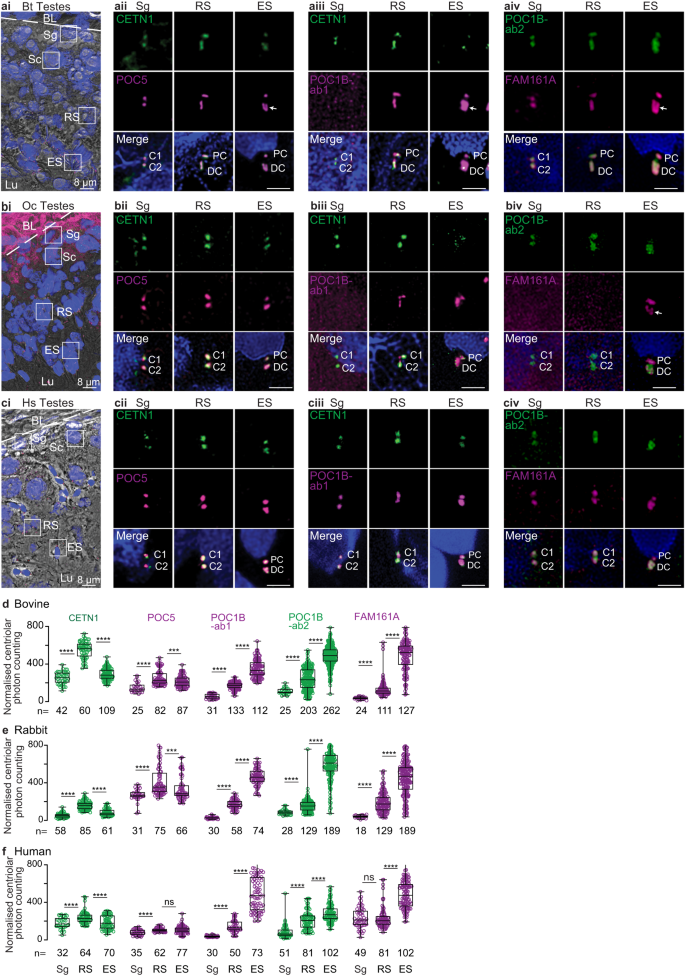
a–a A single seminiferous tubule section showing various stages of spermatogenesis in bovines (ai), rabbits (bi), and humans (ci). The white dotted line indicates the basal lamina boundary. Throughout the paper: BL basal lamina, Sg spermatogonia, Sc spermatocyte, RS round spermatid; Es elongated spermatid, Lu lumen. Scale bars are 8 μm. Representative images of various rod proteins at various stages of spermatogenesis in bovines (aii–iv), rabbits (bii–iv), and humans (cii–iv). Scale bars are 2 μm. Quantification of total centriolar localization, including various proximal and distal centriolar proteins at various stages of sperm development in bovines (d), rabbits (e), and humans (f). The data was generated from three independent experiments. C1/2, centrioles 1 and 2; PC proximal centriole, DC distal centriole, Bt Bos Taurus (bovine), Oc Oryctolagus cuniculus (rabbit), Hs Homo sapiens (human), n, sample size. The two centrioles in Sg are labeled as C1 and C2 since the proximal and distal centrioles are not phenotypically distinguishable at this stage. The white arrow marks the “V”-shaped rods or filled-in “V” shape. The graphs are presented as box and whisker plots, where upper and lower bounds show interquartile range, the line within the box shows the median, and whiskers show minimum and maximum data points. ****P < 0.0001, ***P < 0.001, **P < 0.01, *P < 0.05 (unpaired, two-tailed t test; exact p-values are provided in the Source Data File); ns not significant, n number of cells. Data shown are the representative images and compiled quantification from at least three independent experiments. Source data are provided in the Source Data File.
In pre-haploid sperm cells, CETN1, POC5, POC1B, and FAM161A co-localized in the two centrioles (Sg in Fig. 6a–c). POC1B was detected only with POC1B antibody 2 (Ab2) in pre-haploid sperm of bovine and rabbits (Sg in Fig 6aiii–iv & Fig 6biii–iv), though this was likely due to a lower reactivity of POC1B antibody 1 (Ab1) in these species and the generally lower immunoreactivity of POC1B at this cell stage (Sg in Fig. 6d–e). Like POC1B, CETN1, POC5, and FAM161A also showed the lowest immunolabeling intensity at this sperm cell stage in all species, suggesting that later stages are characterized by enrichment of all four proteins (Sg in Fig. 6d–f). Additionally, the length of CETN1 labeling across all three species was similar (~310 nm), suggesting that before centriole remodeling, the centriolar inner scaffold has an evolutionarily conserved size (Supplementary Fig. 11).
In round spermatids, CETN1, POC5, POC1B, and FAM161A labeling increased in length in the two centrioles (RS in Fig. 6a–c). Accordingly, the labeling intensity of all four proteins was higher at this stage than at the earlier pre-haploid stage in all three species (RS in Fig. 6d–f). In rabbits, FAM161A labeling was undetectable, which was likely due to a weaker immunoreactivity of the FAM161A antibody in this organism. In all three species, CETN1 labeling in the round spermatid proximal and distal centrioles was longer than in pre-haploid sperm centrioles, and distal centriole labeling was longer than that of the proximal centriole (Supplementary Fig. 11). The length of CETN1 in the round spermatid proximal and distal centrioles was species-specific, being longest in bovine (451 ± 62 and 660 ± 72 nm, respectively), intermediate in rabbit (409 ± 40 and 477 ± 72 nm, respectively), and shortest in humans (356 ± 59 and 378 ± 46 nm, respectively) (Supplementary Fig. 11). Together, these findings in round spermatids suggest that the first stage of remodeling and enrichment of the four rod proteins in the proximal and distal centrioles is conserved, but the size extension is species-specific.
In elongated spermatids, CETN1 and POC5 immunostaining intensity levels in the two centrioles were maintained or declined relative to their levels in round spermatids in all three species (ES in Fig. 6d–f). In contrast, POC1B and FAM161A showed more intense labeling in elongated spermatids than in round spermatids of all three species (ES in Fig. 6d–f), while CETN1 showed an inconsistent staining pattern (ES in Fig. 6a–c). Proximal centriole labeling by the four proteins (which was extended in round spermatids) was shortened in elongated spermatids. In the elongated spermatid distal centriole, POC5 reorganized into a “V”-shape in bovine and a wide focus in rabbits and humans (ES in Fig. 6a–c). POC1B labeling took on a filled “V”-shape in the distal centriole of bovine elongated spermatids and a wide focus in the distal centriole of rabbit and human elongated spermatids (ES in Fig. 6a–c). FAM161A labeling reorganized into a “V”- shape in the distal centriole of bovines and rabbits and into a wide focus in humans (ES in Fig. 6a–c). Together, these findings suggest that the second stage of remodeling is also conserved and includes shortening toward their original length of the four rod proteins in the proximal centriole and the formation of a wider distribution and appearance of two rods in the distal centriole.
Quantification of CETN1, POC5, POC1B, and FAM161A in individual proximal and distal centrioles during bovine spermatogenesis found localization intensity changes that are similar to what is observed during total centriole quantification (Supplementary Fig. 12).
Overall, the spermatogenesis findings demonstrate that rod proteins share two conserved remodeling steps with some species-specific length differences. This conservation in distantly related mammalian groups suggests that this program is ancestral in mammals. As part of this program, FAM161A is gradually recruited to the proximal and distal centrioles during spermiogenesis.
The bovine distal centriole begins splaying in round spermatids and is asymmetric in elongated spermatids
This remodeling program may have additional characteristics that are difficult to appreciate with confocal microscopy alone. Therefore, we next performed STORM microscopy in bovine to better understand the organization of centriole remodeling.
Among the species we studied, bovines had the longest round spermatid centrioles. Therefore, we used high resolution N-STORM microscopy to collect more detailed information about their proximal and distal centrioles (Supplementary Fig. 13a). We confirmed that the round spermatid proximal centriole is longer than the pre-haploid proximal centriole and that the round spermatid distal centriole is longer than the round spermatid proximal centriole (Supplementary Fig. 13b). We noticed that the rostral end of the round spermatid distal centriole was 20–23% wider than its caudal tip, as labeled by CETN1, POC5, and POC1B (Supplementary Fig. 13c), suggesting that the distal centriole begins splaying in parallel to rod protein extension in round spermatids. Note that at the round spermatid stage, the proximal centriole elongates to form the centriolar adjunct, which is a short axoneme-like structure that appears during spermiogenesis110,111; this indicates that the rod proteins may extend into the centriolar adjunct. When elongated spermatids are oriented such that the proximal centriole is on the right side, we observed, using STORM, that the left rod of the bovine distal centriole is longer and wider than the right rod, indicating that distal centriole asymmetry arises as part of centriole remodeling during spermiogenesis (Supplementary Fig. 13d). Altogether, these findings suggest that the distal centriole begins splaying in round spermatids and that rod asymmetry is apparent in elongated spermatids.
Deer mice followed the ancestral centriole remodeling program, but house mice pivoted to a centriole degradation program
The primary structure and spermatozoan localization of FAM161A suggest that the family Cricetidae has intermediately modified sperm centrioles. Therefore, we next analyzed centriolar inner scaffold protein localization during spermatogenesis in deer mice (Peromyscus maniculatus), a Cricetidae species with a recognizable proximal centriole and modified FAM161A. We found that CETN1, POC5, POC1B, and FAM161A labeling in deer mouse spermatids was consistent with the ancestral remodeling program observed in the basal mammals (i.e., bovines, rabbits, and humans) (Fig. 7a, b). This finding supports the hypothesis that Cricetidae species undergo a remodeling program that is similar to most other mammals.
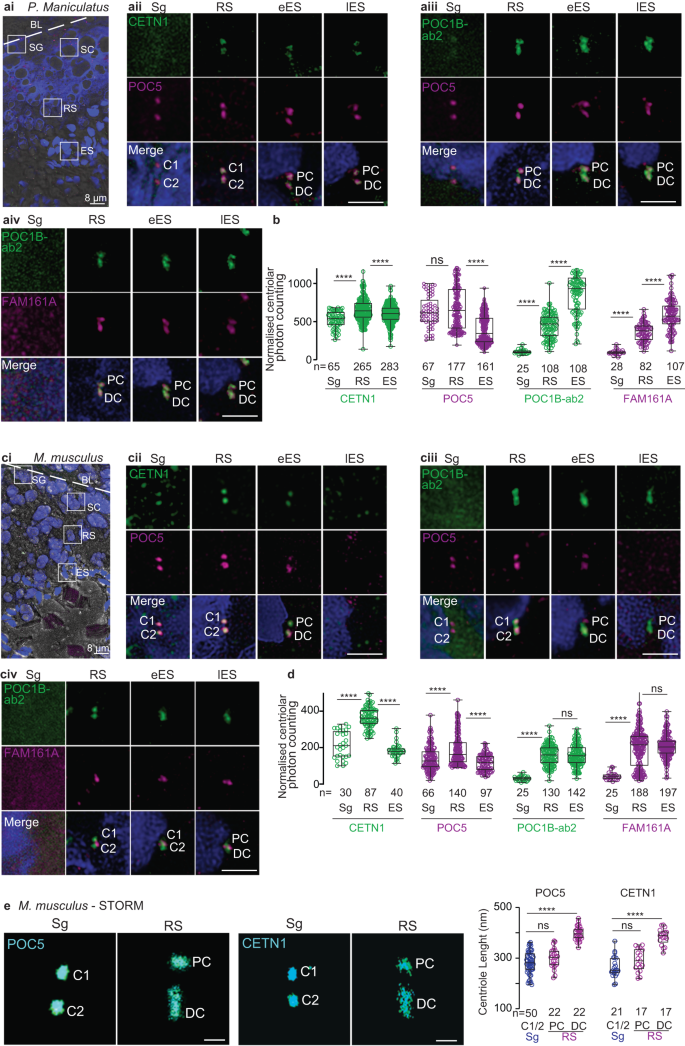
a, c A single seminiferous tubule section showing various stages of spermatogenesis in deer mice (ai) and house mice (ci). The white dotted line indicates the basal lamina boundary. BL basal lamina, Sg spermatogonia, Sc spermatocyte, RS round spermatid, Es elongated spermatid, Lu lumen. Scale bars are 8 μm. Representative images of various rod proteins at various stages of spermatogenesis in deer mice (aii–iv) and house mice (cii–iv). b, d Quantification of the combined proximal and distal centriolar localization of various proteins at various stages of sperm development in deer mice (b) and house mice (d). e STORM imaging of house mouse testes with POC5 and CETN1 staining in spermatogonia/spermatocytes (Sg) and round spermatids (RS) (left panels). Measurements of centriolar length as determined using STORM imaging (right panel). Scale bars are 0.4 μm. The data were generated from three independent experiments; n number of cells in b and d, and number of centrioles in e. The graphs are presented as box and whisker plots, where upper and lower bounds show interquartile range, the line within the box shows the median, and the whiskers show minimum and maximum data points. ****P < 0.0001, ***P < 0.001, **P < 0.01, *P < 0.05 (unpaired, two-tailed t-test; exact p-values are provided in the Source Data File). Source data are provided in the Source Data File.
Next, we analyzed centriolar inner scaffold protein localization during spermatogenesis in house mice (Fig. 7c–e and Supplementary Fig. 14). House mouse spermatozoa contain two highly modified centrioles (remnant centrioles) that are characterized by the lack of CETN18,24, a proximal centriole comprised of a few doublet or triplet microtubules, and a distal centriole comprised of the central pair56.
In house mouse pre-haploid sperm cells, like in bovines, rabbits, humans, and deer mice, CETN1 and POC5 co-localized in the two centrioles (Sg in Fig. 7cii). POC1B and FAM161A were undetected in house mouse pre-haploid sperm cells (Sg in Fig 7ciii–iv), which was likely due to the antibodies having a weaker immunoreactivity in house mice than in other species and to the levels of these proteins being lower at this sperm cell stage than at other stages (Sg in Fig. 7cii–iv and Fig. 7d).
In house mouse round spermatids, like in bovines, rabbits, and humans, CETN1, POC5, and POC1B labeled the proximal and distal centrioles (RS in Fig. 7cii–iii), and the length of the labeling was longer in the distal centriole than in the proximal centriole and pre-haploid centrioles (Fig. 7e). Also, like in bovines, rabbits, and humans, the labeling intensity of the three proteins was higher in round spermatid centrioles than in earlier, pre-haploid sperm cells (Fig. 7d). Unlike in bovines, rabbits, humans, and deer mice, house mouse FAM161A was present as one or two dot-like structures near the proximal centriole-distal centriole junction (RS in Fig. 7civ), and a similar labeling pattern was observed using a second FAM161A antibody (Supplementary Fig. 14). Together, these round spermatid findings suggest that most aspects of the first stage of centriole remodeling in house mice (centriolar elongation and enrichment of CETN1, POC5, and POC1B in the distal and proximal centrioles) are similar to those observed in other mammals.
In house mouse elongated spermatids, rod protein localization was different between early and late elongated spermatids; this difference was not observed in bovines, rabbits, and humans. Like in bovines, rabbits, nor humans, CETN1 and POC5 were present in house mouse early elongated spermatids (eES in Fig. 7cii–iii); however, unlike in bovines, rabbits, and humans, CETN1 and POC5 were undetectable in the centrioles of house mouse late elongated spermatids. (lES in Fig. 7cii–iii). Also, distinct from bovines, rabbits, humans, and deer mice, POC1B and FAM161A were not enriched in elongated spermatids relative to round spermatids. Rather, FAM161A was observed as a pair of dots between the POC1B-labeled proximal and distal centrioles in late elongated spermatids (eES & lES in Fig. 7civ). Together, these findings in house mouse elongated spermatids suggest that the second centriole remodeling stage (centriolar enrichment of POC1B and FAM161A) is dramatically modified in house mice and consistent with a reduction in rod proteins.
Overall, our findings during spermatogenesis reveal that, in general, centriolar inner scaffold proteins in house mouse spermatids begin the remodeling process like in other mammalian species but then pivot to becoming reduced in elongated spermatids. This pivot suggests that the house mouse undergoes a derived remodeling program called centrosome reduction (i.e., degradation)112, which appears to lead to the absence of sperm centrioles in this organism. In this centrosome reduction (i.e., degradation) program, FAM161A labels only one structure found at the proximal centriole-distal centriole junction.
Quantification of CETN1 and POC5 in individual proximal and distal centrioles during house mouse spermatogenesis found localization intensity changes that are similar to what is observed during total centriole quantification (Supplementary Fig. 15).
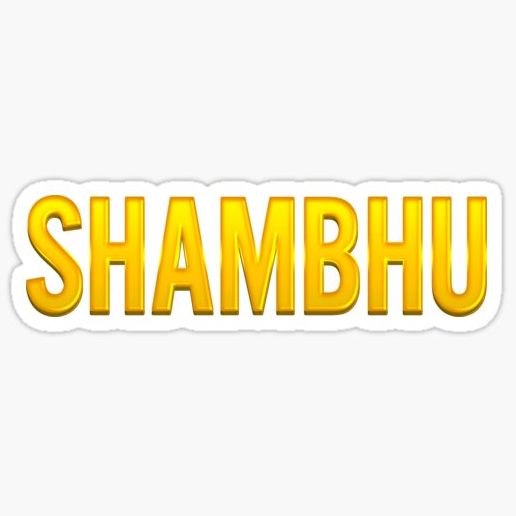
Shambhu Kumar is a science communicator, making complex scientific topics accessible to all. His articles explore breakthroughs in various scientific disciplines, from space exploration to cutting-edge research.